Have you ever wondered how traits are passed down from one generation to the next? You’re not alone. For centuries, people have observed patterns of inheritance, but it wasn’t until the mid-1800s that Gregor Mendel, a monk with a green thumb, cracked the code. Through meticulously planned experiments with pea plants, Mendel unveiled the fundamental principles of heredity, laying the groundwork for modern genetics.
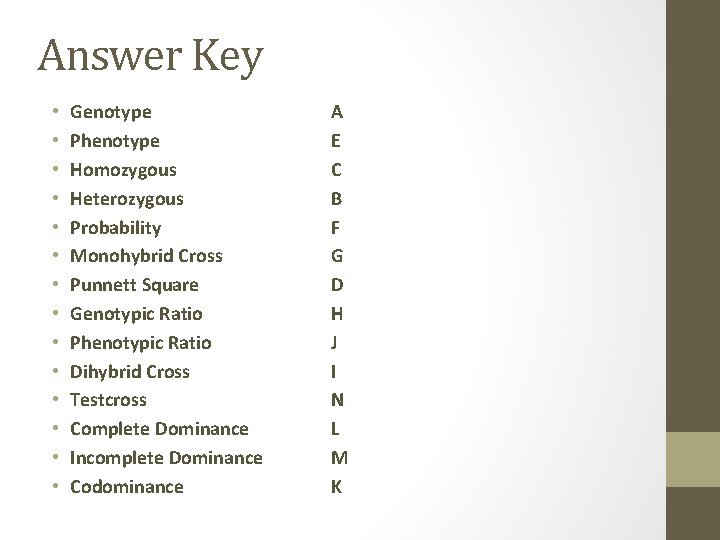
Image: markirishwork.blogspot.com
One of Mendel’s key findings was that certain traits, like flower color or seed shape, are passed down in predictable patterns. These observations led him to propose the existence of “factors” that determined these traits, called genes today. These genes exist in pairs, one inherited from each parent, and they can have alternate forms known as alleles. A monohybrid cross, as introduced by Mendel, is a controlled breeding experiment that focuses on the inheritance of a single trait, such as flower color.
Unveiling the Secrets of Inheritance: A Step-by-Step Look at Monohybrid Crosses
1. Setting the Stage: Defining Terms
Before diving into the mechanics of a monohybrid cross, let’s clarify some essential terms:
- Genotype: The genetic makeup of an organism, representing the combination of alleles for a specific trait. It’s like the blueprint of a trait, determining its potential expression.
- Phenotype: The observable characteristics of an organism, resulting from the interaction of its genotype and the environment. It’s the physical manifestation of the coded instructions within the genes.
- Dominant Allele: An allele that masks the expression of its recessive counterpart when present. Think of it as the more assertive member of the allelic pair, controlling the trait’s appearance.
- Recessive Allele: An allele whose expression is masked by the dominant allele. It’s only visible in the phenotype if two copies of the recessive allele are present.
- Homozygous: Having two identical alleles for a specific trait. It’s like having two copies of the same design instructions, leading to a predictable outcome.
- Heterozygous: Having two different alleles for a specific trait. It’s like having two different design instructions, leading to a potential for variation in the expression of the trait.
2. The Parent Generation (P): The Seeds of Variation
Imagine two pea plants, one with purple flowers and the other with white flowers. These plants represent the Parental generation (P). Let’s assume that purple flower color is dominant (P) and white flower color is recessive (p). The purple-flowered parent plant would be homozygous dominant (PP), while the white-flowered parent plant would be homozygous recessive (pp).

Image: frogedu-book.blogspot.com
3. The First Filial Generation (F1): Blending the Inheritance
When these parent plants are crossed, they produce offspring called the First Filial generation (F1). Each parent contributes one allele for flower color. The purple-flowered parent (PP) can only contribute a P allele, while the white-flowered parent (pp) can only contribute a p allele. Therefore, all F1 offspring will be heterozygous (Pp), carrying one dominant P allele and one recessive p allele.
Since purple is dominant, all F1 offspring will have purple flowers, despite carrying the recessive allele for white flowers. This phenomenon is known as **complete dominance**, where the dominant allele fully masks the recessive allele’s expression.
4. The Second Filial Generation (F2): Recessiveness Unveiled
The F1 generation is then allowed to self-fertilize, producing the Second Filial generation (F2). When two heterozygous plants (Pp) are crossed, we get a different outcome. Each parent has a 50% chance of contributing a P allele and a 50% chance of contributing a p allele.
To visualize the possible outcomes, we employ a tool called a Punnett square. This diagram helps us predict the possible genotype combinations and their corresponding phenotypes in the offspring. In our case, the Punnett square for F2 reveals the following genotype ratios:
- PP: 1/4
- Pp: 1/2
- pp: 1/4
The corresponding phenotype ratio is also 3:1, with 3/4 of the F2 plants exhibiting purple flowers and 1/4 exhibiting white flowers. This reappearance of the recessive white flower phenotype in the F2 generation beautifully illustrates Mendel’s findings about the inheritance of traits.
5. Beyond the Basics: The Power of Monohybrid Crosses
Mendel’s monohybrid crosses laid the foundation for our understanding of inheritance. These simple experiments are not just theoretical exercises; they have practical applications in various fields:
- Agriculture: Understanding monohybrid crosses allows breeders to selectively cross plants to enhance desirable traits, such as yield, disease resistance, and nutritional content. This has been instrumental in improving crop production and food security globally.
- Medicine: Monohybrid crosses are used to study the inheritance of genetic disorders such as cystic fibrosis, sickle cell anemia, and Huntington’s disease. This knowledge allows for genetic counseling and personalized medicine, improving healthcare outcomes. Researchers are even using genetic engineering techniques to develop disease-resistant crops, offering potential solutions to global health challenges.
- Animal Breeding: Similar to agriculture, monohybrid crosses are applied in animal breeding to enhance desirable traits. For example, breeders can selectively breed cattle for high milk production or dogs for specific temperaments and physical characteristics. Ethical considerations, however, are paramount in animal breeding practices, ensuring the well-being of the animals.
Monohybrid Cross Practice Give Peas A Chance
The Legacy of Peas: A Lesson in Heredity
With their simple structure and easily observable traits, pea plants proved to be the perfect model system for Mendel’s groundbreaking studies. His experiments, carefully designed and meticulously analyzed, unveiled the fundamental principles of inheritance, providing a foundation for modern genetics. The legacy of peas continues to inspire research in fields ranging from agriculture to human health, demonstrating the profound impact of understanding the basic mechanisms of heredity.
By carefully observing the patterns of inheritance in simple monohybrid crosses, Mendel illuminated the hidden world of genes and alleles, providing a framework for understanding the complexities of life. From a small plot of peas, a revolution in biology bloomed, transforming our understanding of how traits are passed down through generations.
So, the next time you see a field of peas, take a moment to appreciate the legacy of Mendel’s experiments and the power of understanding monohybrid crosses. We have come a long way from simple observations of peas, but the fundamental principles of inheritance continue to guide our understanding of life’s incredible diversity.